In classical mechanics, two identical particles can always be distinguished, at least in principle. If we know the position of the two particles at some instant t0, then at any time t > t0 we can assign an exact position to each of them by simply calculating their trajectory. In quantum mechanics, however, this possibility is prevented by the uncertainty relations, which imply that the more precisely the initial position of a particle is known, the larger is the spread of its velocity. This means that, even though the position of a particle is known accurately at a given instant, what we will have shortly afterwards is a probability distribution. Obviously, as soon as the distributions of two particles overlap, their position can no longer work as a label. We say therefore that in quantum mechanics two identical particles are, in general, indiscernible.
Because of its symmetry properties under permutation of particle labels, an ensemble of identical quantum particles can only be found in either a symmetric or antisymmetric quantum state. (This constraint does not apply to distinguishable quantum systems, e.g. atoms in a crystal lattice, whose quasi-fixed position acts as a label.) In the former case the particles are called bosons (in honor of Satyendra Nath Bose), in the latter case they are called fermions (in honor of Enrico Fermi).
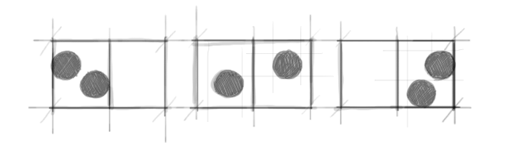
Figure 1. Comparison of the Boltzmann, Bose and Fermi distributions for the case of two particles and two equiprobable individual states (represented by the two cells).
BOLTZMANN : | 1/4 | 1/3 | 1/4 |
BOSE : | 1/2 | 1/3 | 1/3 |
FERMI : | 0 | 1 | 0 |
Integer spin particles always behave like bosons. Half-integer spin particles always behave like fermions. Photons and mesons are examples of bosonic elementary particles; electrons, neutrinos and quarks are all fermions. Compound systems, like atoms and ions, have a fermionic or bosonic character depending on their total 'spin (calculated by adding the spins of their parts).
The most important consequences of the indiscernibility principle are found in the physics of aggregates. Indeed, the statistical behavior of an ensemble of identical particles is radically different depending on whether particles are distinguishable or indistinguishable.
The statistical approach to ensembles of distinguishable particles was developed by Wolfgang Boltzmann in his kinetic theory of ideal gas. For ensembles of indistinguishable particles, there exist two kinds of statistics: the Bose-Einstein statistics for bosons and the Fermi-Dirac statistics for fermions. The two display very different features at low temperatures.
A peculiar feature of bosons is that they like to keep together: N bosons already in a quantum state will enhance the probability of one more joining them. The opposite is true for fermions, which must obey Pauli's exclusion principle. In the table above we consider the case in which a two-value observable with equiprobable outcomes is measured on a pair of identical particles (the right and left halves of the boxes represent the two states accessible to the particles, each corresponding to an eigenstate of the observable). The toss of two coins provides a good model for such a situation. If we compare the statistical distributions expected for a pair of bosonic coins, a pair of fermionic coins and a pair of distinguishable (classical) coins, we see that using fermionic coins one never gets two tails nor two heads, whereas with bosonic coins this happens more likely than it does with distinguishable coins.