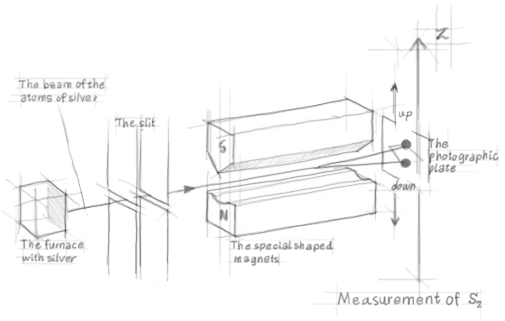
Figure 1. Stern-Gerlach Experiment.
Because of their electronic structure, silver atoms have a global spin that can be roughly identified with the spin of the electron orbiting in the outer electronic shell. If a beam of silver atoms is directed through an inhomogeneous magnetic field with vertical gradient, it splits in two well-separated components along the z axis (see figure 1). This is exactly the behavior expected for two ensembles of atoms that have angular momenta equal in modulus but pointing in opposite directions (like tops spinning either clockwise or counterclockwise with the same angular velocity).The same split along the axis parallel to the gradient of the magnetic field is observed if the experiment is repeated by changing the orientation of the magnets. Thus, what we find is that the spin component along any direction can only take two values that we conventionally call spin up and spin down.
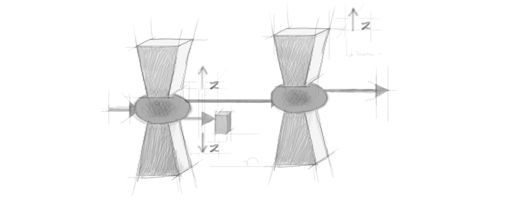
Figure 2. Selection of Sz 'up'.
The incompatibility of two orthogonal components of spin, for example Sz and Sx, shows up when we try to prepare the atoms in such a way that a joint measurement of Sz and Sx should yields up-up with probability 1, i.e. p(Sz= ↑ & Sx= ↑ )=1. Figure 2 shows the filtering process through which we prepare atoms for which p(Sz= ↑). An analogous filter (with the magnets oriented perpendicularly) can be used to prepare atoms for which p(Sx= ↑).The problem is that if we try to implement a double filtering process in order to prepare atoms for which p(Sz= ↑ & Sx= ↑)=1 (figure 3), it simply doesn't work! When exiting the Sx filter, atoms display Sz= ↑ or Sz= ↓ with equal probability (and not, as could be expected in a deterministic model, Sz= ↑ with probability 1).
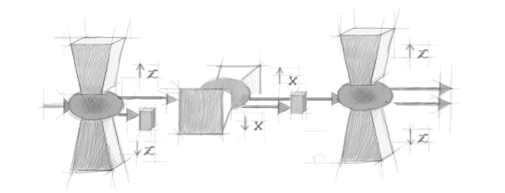
Figure 3. Double filtering process.
This result indicates that the probability associated with the different outcomes in two successive measurements of Sz is modified depending on whether Sx is measured or not in between. It is important to stress that this modification occurs independently of the set-up used to perform the measurement, i.e. it is not brought about by the particular nature of the interaction between the atoms and the Stern-Gerlach apparatus. Rather, it is a typical feature of measurements involving two observables that are mutually incompatible. As we show in the section on origins, this odd situation can be easily represented by means of state vectors within the state space.The contrast between deterministic models (see hidden variables) and the quantum formalism involving incompatible observables becomes even sharper when we consider the correlations that exist between pairs of entangled particles. Experiments with entangled pairs (and also with triplets and quadruplets of entangled particles) have been carried out using two-level systems analogous to spin up/spin down particles.
The polarization of photons provides for example a close analogous of electron spin. If one directs a light beam through polarizing filters (that play the role of the Stern-Gerlach apparatus), the observed results are equivalent to those described in this section. Classical electromagnetism can account for observations in this case. However, when the same optical experiments are carried out with individual photons, a quantum treatment that makes essential use of state vectors becomes necessary (see also particle interference).
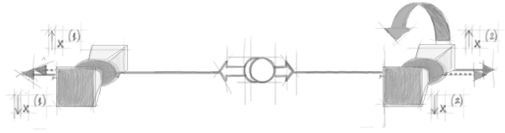
Figure 4. The correlations between the spins of two entangled particles are measured by means of two distant Stern-Gerlach apparatuses. The relative orientation of the two apparatuses can be changed. When they are parallel, the spins of the two particles are always found to point in opposite directions (see 'origins' for further discussion).